In vitro Modelling of the Gut Brain Axis and Microbiome for Neurodegenerative Diseases
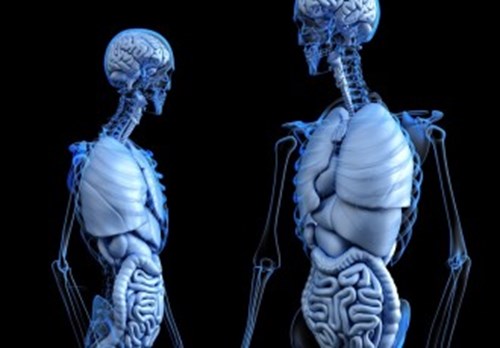
The human microbiome is a complicated ecosystem. It forms a large population that can be considered as a symbiotic super-organism in combination with the human body. There are 10 times more microbial cells than Homo sapiens cells in the body and the human microbiome, which contains millions of genes, has a greater protein-coding potential than the entire human genome, which contains only 20 000 or so genes1. The gut microbiome is essential to healthy neuronal development and it has been shown that germ-free upbringing of mice induces significant permanent neurodevelopmental deficits2.
The gut-brain axis (GBA) (See Figure 1) involves two-way communication between the emotional and cognitive centres of the brain with and nerves of the intestines. It is now generally accepted that there is an influential role of the gut microbiome on the GBA through neural, endocrine, immune, and humoral signalling. Clinical evidence for this comes from the association microbiome imbalance in autism, anxiety-depressive disorders and gastrointestinal disorders such as irritable bowel disease3 and more recently neurodegenerative diseases. There is growing evidence of a GBA/microbiome involvement in Parkinson’s Disease, which is usually displays motor impairment and non-motor gastrointestinal dysfunction and inflammation in the enteric nervous system4. Similar associations have been found in multiple sclerosis5 where there are imbalances in the gut microbiome6 and some therapeutic benefit has been demonstrated by foecal microbiome transplant7. Most recently a relationship between microbiome dysfunction has been made with the progression of Alzheimer’s disease8.
Animal and in vitro models (including the cell line SH-SY5Y) have been used to investigate the effect on neurological disorders such as anxiety using the bacterium Bifidobacterium longum9 and it seems likely that there will be a future need for advanced in vitro mechanistic co-culture models for the study of microbiome interactions with the GBA in neurodegenerative diseases.
Models of the gut microbiome are already being developed, either with10 or without11 the presence of an intestinal epithelial component. In vitro cell culture models of the intestine are already established in drug discovery and development and their suitability for use in the field of food microbiology have been reviewed12. Recently a cellular model based on human colon carcinoma CACO-2, HT29 cells, and Raji cells was established to provide a model that more accurately mimics the intestinal epithelium13. To achieve a model that recapitulates all aspects of the GBA will have to incorporate not only neuronal, intestinal epithelial, endothelial, and stromal cell types but also immune cells (such as the co-culture with Raji B cells13) and more complex immune models14 in order to achieve a physiological state of homeostasis. It is also highly likely that intestinal models developed from induced pluripotent stem cells (iPSC)15 16 could be developed for GBA/microbiome studies.
With its extensive collections of authenticated cell cultures and iPSC cell lines (ECACC), bacteria and mycoplasmas (NCTC) pathogenic viruses (NCPV) and fungi (NCPF) the Culture Collections of Public Health England provide a unique resource for any researches involved in the in vitro modelling of the GBA and microbiome.
Figure1: The Structure of the Microbiome Gut Brain Axis.
The central nervous system, specifically the hypothalamic-pituitary-adrenal axis (dotted lines) is activated in response to emotion and stress. Cortisol is released and driven by interactions between the amygdala (AMG), hippocampus (HIPP) and the hypothalamus (HYP) (together constituting the limbic system). Corticotropin-releasing factor (CRF) is released by the hypothalamus stimulating adenocorticotopic hormone (ACTH) secretion by the pituitary gland leading to cortisol release by the adrenal glands. In parallel the central nervous system communicates along the afferent and efferent autonomic pathways with the enteric nervous system of the gut which in turn modulate mucus secretion, motility, immunity and permeability. The microbiome is in two-way communication with this system3.
References
1. Gundogdu, A. & Nalbantoglu, U. Human genome-microbiome interaction: metagenomics frontiers for the aetiopathology of autoimmune diseases. Microb. Genomics3, (2017).
2. Luczynski, P. et al. Growing up in a Bubble: Using Germ-Free Animals to Assess the Influence of the Gut Microbiota on Brain and Behavior. Int. J. Neuropsychopharmacol.19, (2016).
3. Carabotti, M., Scirocco, A., Maselli, M. A. & Severi, C. The gut-brain axis: interactions between enteric microbiota, central and enteric nervous systems. Ann. Gastroenterol. Q. Publ. Hell. Soc. Gastroenterol.28, 203–209 (2015).
4. Perez-Pardo, P. et al. The gut-brain axis in Parkinson’s disease: Possibilities for food-based therapies. Eur. J. Pharmacol.817, 86–95 (2017).
5. Ghaisas, S., Maher, J. & Kanthasamy, A. Gut microbiome in health and disease: Linking the microbiome–gut–brain axis and environmental factors in the pathogenesis of systemic and neurodegenerative diseases. Pharmacol. Ther.158, 52–62 (2016).
6. Jangi, S. et al. Alterations of the human gut microbiome in multiple sclerosis. Nat. Commun.7, 12015 (2016).
7. Smits, L. P., Bouter, K. E. C., Vos, W. M. de, Borody, T. J. & Nieuwdorp, M. Therapeutic Potential of Fecal Microbiota Transplantation. Gastroenterology145, 946–953 (2013).
8. A. Kohler, C. et al. The Gut-Brain Axis, Including the Microbiome, Leaky Gut and Bacterial Translocation: Mechanisms and Pathophysiological Role in Alzheimer’s Disease. Curr. Pharm. Des.22, 6152–6166 (2016).
9. Bercik P. et al. The anxiolytic effect of Bifidobacterium longum NCC3001 involves vagal pathways for gut–brain communication. Neurogastroenterol. Motil.23, 1132–1139 (2011).
10. Bahrami, B., Child, M. W., Macfarlane, S. & Macfarlane, G. T. Adherence and Cytokine Induction in Caco-2 Cells by Bacterial Populations from a Three-Stage Continuous-Culture Model of the Large Intestine. Appl. Environ. Microbiol.77, 2934–2942 (2011).
11. Venema, K. & van den Abbeele, P. Experimental models of the gut microbiome. Best Pract. Res. Clin. Gastroenterol.27, 115–126 (2013).
12. Cencič, A. & Langerholc, T. Functional cell models of the gut and their applications in food microbiology — A review. Int. J. Food Microbiol.141, S4–S14 (2010).
13. Antunes, F., Andrade, F., Araújo, F., Ferreira, D. & Sarmento, B. Establishment of a triple co-culture in vitro cell models to study intestinal absorption of peptide drugs. Eur. J. Pharm. Biopharm.83, 427–435 (2013).
14. Sardi, M., Lubitz, A. & Giese, C. Modeling Human Immunity In Vitro : Improving Artificial Lymph Node Physiology by Stromal Cells. Appl. Vitro Toxicol.2, 143–150 (2016).
15. Watson, C. L. et al. An in vivo model of human small intestine using pluripotent stem cells. Nat. Med.20, 1310–1314 (2014).
16. Spence, J. R. et al. Directed differentiation of human pluripotent stem cells into intestinal tissue in vitro. Nature470, 105–109 (2011).